Air humidity in rooms – thermodynamics and enthalpy
Introduction
What is “moist” air, when or why does it become “dry”, how much heat energy does it contain, and what does all this have to do with specific enthalpy? An insight into the correlations of air humidity in rooms is provided by physics, more precisely by the laws of thermodynamics. Anyone who has grasped this not entirely simple subject knows, for example, about possible ways of playing with the dewpoint of water in evaporative coolers, can explain the concept of wet bulb temperature without a second thought, and has an impressive grasp of isentropic changes of state – not to mention, being able to sleepwalk through the h-x diagram with complete accuracy, even without an explanatory video as back-up.
These days, however, the design of air conditioning units, HVAC systems or recooling systems is very often carried out by computer programs. The danger of this: Expert knowledge gained by planners or plant engineers about the thermodynamic behaviour of air humidity is increasingly being forgotten. And sometimes there is simply not enough time in day-to-day business to give any thought to an energy-efficient alternative. However, maybe by thinking about air conditioning technology or recooling units in more depth, one or two kW of mechanically generated cooling energy might be saved or a refrigeration system might even become superfluous? This is especially true when it comes to cooling temperature limit ranges. That is, providing that the possibilities of adiabatic cooling, but also new ones for moisture recovery are properly understood. This is where thermodynamic knowledge helps to deal “correctly” with air humidity in rooms.
Human physiology
Unlike with temperature, we humans are not able to feel changes in relative humidity immediately, only its effects. If it is too low (well below 30% relative humidity), our mucous membranes and skin start to feel dry after a while. If it is too high, on the other hand, we perceive it as feeling muggy and at some point we start to perspire uncomfortably. A healthy body temperature is around 37 °C. As ambient temperatures are usually lower, we give off heat continuously. This is the second law of thermodynamics, which states the reverse: “Heat never transfers on its own from a colder body to a warmer body.” This means that our body's own heat transfer is based on the principles of physics. through “conduction” when we come into contact with colder surfaces, through “convection” when air passes over our skin or through “radiation exchange” between surfaces of different temperatures.
In addition to our clothing, however, we regulate our temperature in another way, i.e. to a large extent through the evaporation of moisture via our largest organ, the skin. This process of evaporation is directly aided by convection processes. When air, which has not yet reached its saturation point, flows over the skin, it can absorb water vapour, which is released by the body. So when our own body heat evaporates the water in our body through the pores and the sweat is absorbed by the air and transported away, we experience this process as heat loss, we feel a change in temperature, we feel cold. When one kilogram of water evaporates through our skin, the body is deprived of just under 700 Wh per hour, in other words about 600 kilo calories. Dry warm air absorbs the water vapour we give off, causing it to evaporate very quickly on the skin. A good example is what happens if we go in a sauna. We can easily withstand a temperature of 90 °C with very low relative humidity because the body can cool itself for a certain amount of time. However, if the relative humidity in the room was 100% at the same temperature as in a steam bath, we would not last a minute and it could even be life-threatening.
Humidity, temperature, pressure and Mollier
In addition to conduction, radiation, convection and clothing, temperature and indoor air humidity therefore play a decisive role for human comfort and well-being. Since both of these variables depend on each other and our bodies rely on them, we even feel the “enthalpy” (abbreviated to “h”) of the air surrounding us to a certain extent. In the field of air conditioning technology, it is used to determine the amount of heat that is added to or removed from the air during a process. This is the same process in our body. It registers the heat content of the air-water mixture that constantly surrounds us. If there are any deviations, we unconsciously try to adapt the heat balance of our bodies to the enthalpy of the indoor air in order to ensure a healthy body temperature. But what happens when temperature and humidity change?
We can all imagine what the state variable of temperature means. However, it is a little more difficult when it comes to the common terms air humidity or air moisture. What is meant is the proportion of water vapour – i.e. not droplets, fog, rain or ice – in the gas mixture in rooms or in the air we breathe. This proportion depends on temperature and air pressure. For example, a certain volume of air can absorb a precisely determinable mass of water vapour, measured in grams of water vapour per kilogram of dry air. This value expresses absolute humidity and is indicated by the symbol “x”. If the air is saturated, it is at the so-called saturation or dewpoint. It is unable to absorb any more water vapour, and the relative humidity “ϕ” is then at 100%. As a matter of fact, this condition hardly ever occurs in nature. This is why the volume percentage of relative water vapour present in the air fluctuates between 0 and 100% when the temperature or pressure changes. This value is of great importance for air conditioning technology. On the one hand, this value, together with the temperature, provides information about the level of comfort in rooms. On the other hand, it allows processes to be calculated and quantities of energy to be determined that are required for evaporation and condensation or that are necessary for evaporation. The correlations are complex, but calculable. This is why computer programs can help in the design of adiabatic and isothermal air humidification processes in rooms or ventilation units. Adiabatic means the atomisation and evaporation of water at a constant temperature. In this process, the smallest water droplets immediately turn into steam and the thermal energy required for this is extracted from the ambient air. A cooling effect occurs. For isothermal humidification, on the other hand, water is heated to boiling point and reaches an enthalpy of h = 419 kJ/kg. At sea level, it then evaporates at 1,013 mbar and 100 °C.
Richard Mollier, Professor of Applied Physics and Mechanical Engineering at the Universities of Göttingen and Dresden in Germany (1897 to 1931), recognised the relationships between the state variables of temperature, absolute and relative humidity, water vapour pressures, enthalpies and densities at the beginning of the 20th century. Without the use of a computer, he combined these with great expertise in the h-x diagram for humid air, which was later named after him. With the help of this diagram, it is still quite easy to depict changes in the state of humid air, such as heating, cooling, humidifying, dehumidifying and mixing, and to quickly read out the thermal power required for air conditioning. Let us consider a simple example to illustrate this.
The effect of evaporative cooling
Fig. 1: Representation of evaporative cooling in the h-x diagram. By humidifying the outside air (32 °C, 40%) to almost 100% humidity, the air temperature drops along the isenthalp by almost 10 K to about 22 °C, i.e. without the supply of evaporation heat (diagonal lines in the diagram). (Fig. cci Dialog GmbH)
Fig. 2: The wet bulb temperature shows maximum possible cooling at the given air temperature and relative humidity, in this case, at sea level. (Fig. cci Dialog GmbH)
Calculating is valuable, but knowledge is worth its weight in gold
Thanks to Mollier's h-x diagram, a specialist planner or ventilation system engineer can check whether a humidification or dehumidification system has been correctly dimensioned. The diagram shown in Fig. 3 can also be applied to “The Squaire” multi-complex at the ICE mainline train station at Frankfurt Airport. The building with 140,000 m2 of total rental space for offices, conference rooms, hotels, retail and catering is equipped with a ventilation and air conditioning system with supply air cooling. The outside air of state 1 (32 °C, 40% relative humidity, h = 62.5 kJ/kg) is drawn into a central supply air conditioning unit where it is filtered. The fresh air is then cooled to supply air (state 2) in two coupled heat exchangers with a closed circuit system (CCS).
The water leaving the CCS heat exchanger in the central exhaust air unit at 21.5 °C is cooled to 13 °C in an intermediate heat exchanger using 10 °C cold water (from a refrigeration unit). This water first flows through heat exchanger 2 and then through heat exchanger 1 in the supply air unit before returning to the central exhaust air unit at a temperature of 28 °C. As a result of this pre-cooling and post-cooling, the outdoor air is cooled and dehumidified from 32 °C to state 2 with 15.5 °C, 10 g/kg humidity and an enthalpy of 40 kJ/kg.
After flowing through further air filters and the fan unit (temperature increased by 1.5 K), the supply air in state 3 has a temperature of 17 °C, a relative humidity of 82% and an enthalpy of h = 41.5 kJ/kg, which is introduced into the rooms of the building. The exhaust air heated to 26 °C (state 4) is then drawn in again by the central exhaust air unit and flows through an adiabatic evaporative cooling system after filtering. At this point, the exhaust air is humidified to almost 100% relative humidity and thus cooled to around 18.5 °C (h = 52 kJ/kg) (state 5). The 18.5 °C air then cools the 28 °C warm water in the exhaust air heat exchanger of the CCS system to a flow temperature of 21.5 °C. Finally, the exhaust air with a temperature of about 26 °C (state 6) flows outdoors as exhaust air.
In winter, the system technology of the ventilation and air conditioning systems remains as before, but several operating parameters are changed: The outside air now has a temperature of -12 °C and a relative humidity of 90%. The target temperature of the supply air processed in the central air conditioning units remains at 17 °C even in winter. The adiabatic evaporative cooling in the exhaust air units is switched off. Instead of the refrigeration unit, heating water (district heating with a flow temperature of 85 °C) is now used to temper the water in the CCS system to a maximum flow temperature of 60 °C for heating the supply air. If the outside air is too dry, it is humidified in the central supply air unit. Incidentally, systems for moisture recovery, such as membrane heat exchangers or sorption rotors, also represent an alternative to active humidification in ventilation units. They extract heat (enthalpy) from the exhaust air and a large part of the air humidity and then feed this into the supply air. The advantage of this is that every gram of water vapour required, which does not have to be generated by a technical process, not only saves energy, but also reduces costs and CO2 emissions. However, this process cannot be regulated as easily as active humidification.
Fig. 3: h-x diagram illustrating supply air (1 to 3) and exhaust air treatment including adiabatic evaporative cooling in summer (4 to 6). The lower part of the figure illustrates the coupling of the supply air and exhaust air units with the circulating compound heat recovery system (Fig. 2C: Content + communication)
Precision measurements
Did you know?
To conclude the Testo expertise series on air humidity in rooms, we have compiled some more facts about the whole subject area. They provide general knowledge, but can also be useful in the course of daily work.
- If 1 litre of water with an initial temperature of 20 °C is evaporated at 100 °C (1,013 mbar), this requires about 700 W of energy.
- In winter, the ambient air has an absolute humidity of about 2 g/kg at -8 °C, with a relative humidity of 100%. If this air heats up to a pleasant 22 °C temperature, only 12% relative humidity remains. This air is far too dry for us humans, so it will need humidifying.
- In summer, on the other hand, the humidity in Germany at 20 °C averages 60% relative humidity and 8.5 g/kg absolute humidity. With a spatial volume of 150 m³, this corresponds to about 2 litres of water in the air. If the temperature drops to 0 °C, for example, when entering a cold storage cell, more than 50% of the water content precipitates as dew or can lead to unwanted ice formation.
Fig. 5: (Source: Information leaflet: “Luftbefeuchtung” [Air humidification]; EnergieSchweiz, Bundesamt für Energie BFE [Swiss Federal Office of Energy], 2016)
- Every healthy person has an air conditioning system of their own, and always humidifies the air they breathe through their mucous membranes in the mouth, nose, throat, trachea and bronchi to the maximum possible relative humidity of 100% before it reaches the alveoli.
- If relative humidity falls below 20%, the immune defence of the mucous membranes fails because their self-cleaning function and the removal of bacteria and viruses slow down and eventually stop completely.
- In contrast, viruses and bacteria can only survive for a few minutes at a relative humidity of around 50%. If this value drops significantly, their survival time increases to up to 2 days and the pathogens encapsulated in a salt crust remain suspended. At values well above 60%, germs can also remain infectious for many hours, but only remain in the air for a short time and sink to the ground.
- When sneezing and coughing, particles or pathogens are hurled out of the lungs via the respiratory tract at hurricane force, sometimes at the speed of sound.
- On average, people spend up to 90% of their time in closed rooms, i.e. in an artificially maintained climate.
- During any activity, people can release between 50 and 200 g of water per hour as moisture. When cooking, washing and showering, up to 1,500 g of water can evaporate. Top athletes can even lose between 2,000 and 3,000 g of water when competing.
- In the workplace, most employees feel that indoor air with a relative humidity of well below 30% is too dry. This is the result of a 2-year study by the Fraunhofer Institute for Industrial Engineering on the significance of humidity in the office.
Fig. 6: Source: “Air humidity in the office workplace”; Fraunhofer Institute for Industrial Engineering and Organisation IAO, Stuttgart, 2014
Are you looking for a reliable way to measure indoor air humidity and moisture?
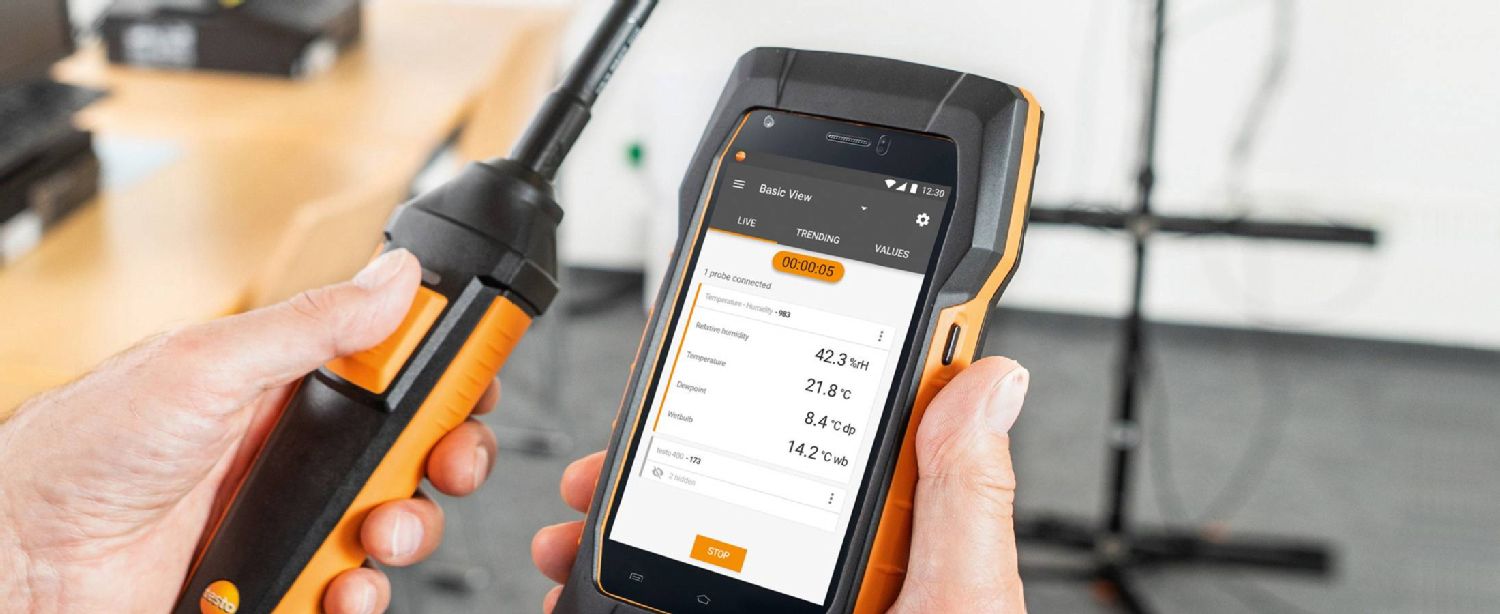